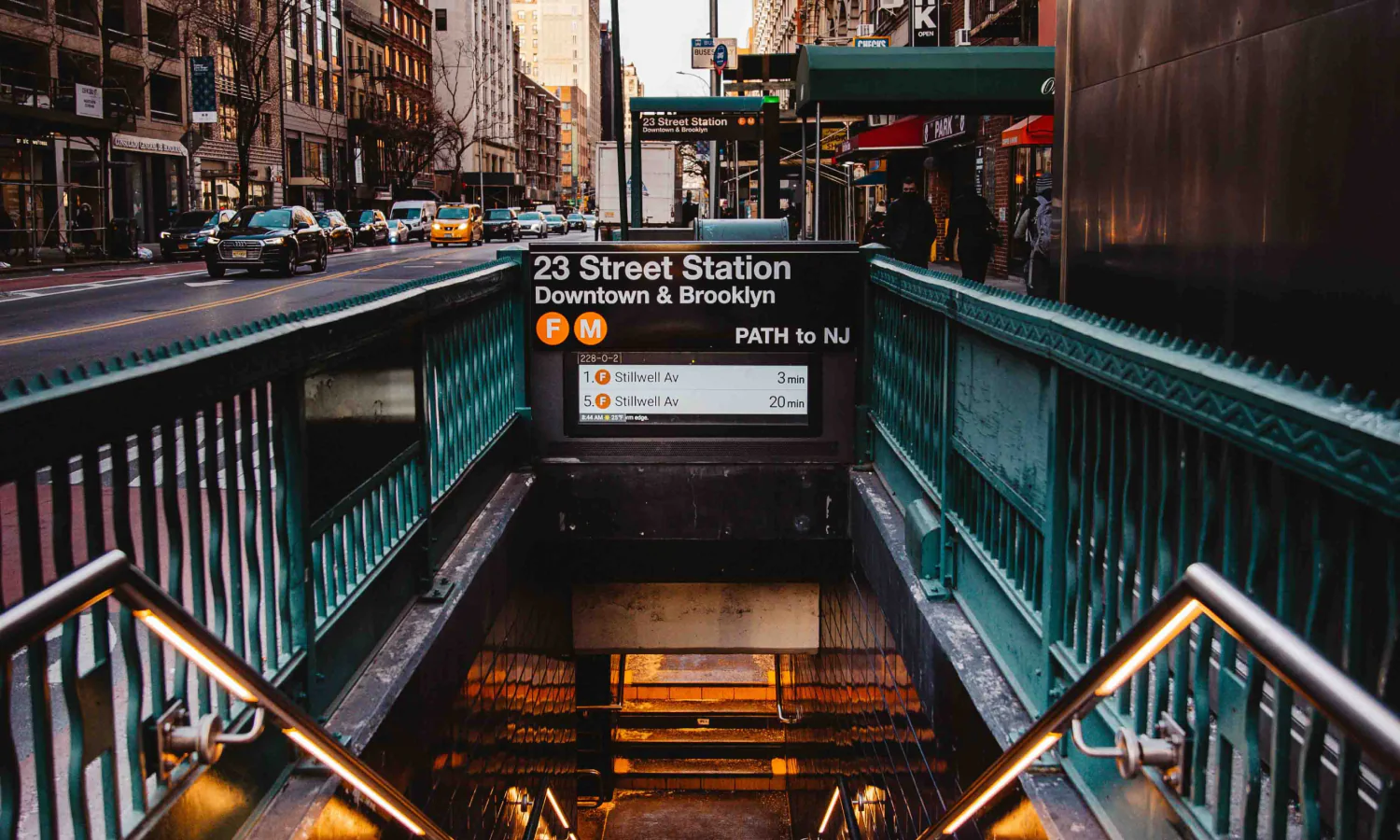
Newsletter Subscribe
Enter your email address below and subscribe to our newsletter
Enter your email address below and subscribe to our newsletter
The P-N junction is a fundamental concept in the field of semiconductors, playing a crucial role in various electronic devices. In this article, we will explore the workings of a P-N junction, including the role of doping, the formation of the depletion region, and the influence of the electric field.
We will also delve into the applications of P-N junctions, the different types, and the factors that affect their performance. We will discuss ways to improve P-N junctions, making this a comprehensive guide to understanding and utilizing this essential component in semiconductor technology.
A P-N junction is a boundary or interface between two types of semiconductor materials, known as the P-type and N-type semiconductors, exhibiting unique electrical properties and applications in modern physics and electronics.
When P-type semiconductor, typically doped with acceptor impurities, comes into contact with N-type semiconductor, doped with donor impurities, a P-N junction forms. This interface creates a depletion region, where electrons from the N-type material migrate to the P-type material, leaving behind positively charged ions on the N-side and negatively charged ions on the P-side, establishing an electric field.
This built-in electric field prevents further migration of charge carriers, resulting in a potential barrier. The fundamental properties of P-N junctions include the rectification of current, allowing the flow of current in one direction and blocking it in the opposite direction, a crucial characteristic for diode functions.
When a forward bias voltage is applied, the barrier is reduced, enabling the conduction of current, while a reverse bias increases the barrier, preventing current flow. The unique electrical properties and behavior of P-N junctions make them fundamental components in various electronic devices, including diodes, transistors, and solar cells, contributing significantly to the advancement of modern technology and semiconductor physics.
The operation of a P-N junction revolves around the movement of electrons and holes due to processes such as diffusion, drift, and recombination, which are essential for the generation of the junction’s unique electrical behavior.
Doping plays a crucial role in the P-N junction by introducing impurities into the semiconductor materials, thereby altering their conductivity and creating distinct N-type and P-type regions essential for the junction’s operation.
The process of introducing impurities through doping is essential for controlling the electrical characteristics of the semiconductor materials.
By adding specific impurities such as boron or phosphorus, the conductivity of the material can be modified, leading to the formation of N-type and P-type regions.
This alteration of conductivity is critical for the functioning of diodes and transistors, as it enables the controlled flow of current across the junction.
The electric field in the P-N junction is responsible for establishing a potential barrier and creating a depletion region, which influences the junction’s behavior under forward and reverse bias conditions, defining its electrical characteristics.
The potential barrier in the P-N junction arises due to the alignment of Fermi levels, leading to the formation of a depletion region that restricts the flow of majority charge carriers.
This depletion region consists of immobile ions, resulting in a built-in electric field that opposes further diffusion of carriers across the junction.
When the junction is under reverse bias, the built-in electric field is strengthened, widening the depletion region and enhancing the barrier for charge carrier movement.
Conversely, during forward bias, the applied external voltage reduces the effective potential barrier, allowing for a significant flow of current through the junction.
The depletion region within the P-N junction acts as a crucial boundary separating majority and minority carriers, contributing to the formation of a barrier potential that influences the junction’s electrical response.
This region is characterized by a lack of mobile charge carriers due to the migration of electrons and holes towards the oppositely charged regions. Minority carriers diffuse across the depletion region, leading to recombination with majority carriers.
This phenomenon plays a pivotal role in restricting the flow of electrical current, thereby defining the junction’s behavior as a rectifier or diode. As a result, the barrier potential forms a critical component influencing the electrical properties of the junction, shaping its application in various electronic devices.
P-N junctions find diverse applications in electronic devices, including diodes, transistors, and solar cells, where their unique properties enable crucial functionalities in modern technology.
P-N junctions serve as the fundamental building blocks of diodes, enabling crucial functionalities such as rectification and regulation of current flow under forward and reverse bias conditions.
When a diode is forward-biased, the P-N junction allows current to flow freely due to the reduced barrier potential. This permits the easy passage of electrons from the N-type material to the P-type material.
On the other hand, under reverse bias, the P-N junction exhibits a significant resistance to the flow of current, effectively blocking its passage. This behavior is vital for ensuring the diode’s efficient operation by controlling the direction and magnitude of current.
P-N junctions play a pivotal role in the functionality of transistors, facilitating amplification and switching operations in various configurations such as NPN and PNP transistors.
These junctions are formed by combining a semiconductor material doped with trivalent (positively charged) atoms with a semiconductor material doped with pentavalent (negatively charged) atoms.
When these materials are brought into contact, a depletion region is formed, creating a barrier potential. This configuration allows for the control of current flow, enabling the transistor to amplify signals or act as a switch in electronic circuits.
P-N junctions are integral to the operation of solar cells, where they facilitate the conversion of sunlight into electrical energy through the generation and collection of electron-hole pairs in photovoltaic devices.
This semiconductor-based mechanism involves the formation of a depletion region at the P-N junction, creating an electric field that accelerates the separation of electrons and holes.
As sunlight strikes the solar cell, it excites the electrons in the P-N junction, allowing them to break free and flow as an electric current. This flow of electrons constitutes the electrical energy harnessed from solar radiation, making P-N junctions a vital component in the photovoltaic conversion process.
P-N junctions exhibit various configurations, including homojunctions, heterojunctions, and Schottky junctions, each showcasing distinct structural and electrical characteristics for specialized applications.
Homojunctions feature P-N junctions formed within the same semiconductor material, exhibiting specific band alignment and junction depth characteristics that influence their electrical behavior and applications.
These junctions are pivotal in semiconductor devices, as they create a boundary between the P-type and N-type semiconductor regions, allowing for the formation of electric fields and charge carrier movement.
The band alignment properties of homojunctions determine the energy barrier at the interface, affecting the device’s electronic properties. The junction depth, defined by the diffusion length of charge carriers, impacts the efficiency of charge separation and collection, critically influencing device performance.
Heterojunctions comprise P-N junctions formed between different semiconductor materials, featuring unique energy band discontinuities and considerations such as lattice mismatch that impact their electrical and optical properties.
These interfaces, created by bringing together different semiconductor materials, exploit their distinct electronic properties to enable advanced device functionalities.
The presence of energy band discontinuities at heterojunctions gives rise to rectification and photovoltaic effects, essential for electronic and optoelectronic applications.
The lattice mismatch between the materials composing a heterojunction influences the formation of defects and strain, affecting the performance of devices.
Engineers and researchers continually explore ways to mitigate the impact of lattice mismatch through innovative material engineering and epitaxial growth techniques to optimize the characteristics of heterojunction-based devices.
Schottky junctions represent P-N junctions formed between a metal and a semiconductor, showcasing unique properties such as barrier height and rectification capabilities crucial for electronic applications.
The composition of Schottky junctions consists of a metal layer in direct contact with a semiconductor material, creating a metal-semiconductor interface that facilitates electron flow in one direction while hindering it in the opposite direction.
The barrier height at this interface determines the energy barrier for electron transport, impacting the junction’s rectification behavior. This feature is fundamental for diode applications where the controlled one-way flow of current is essential for efficient operation.
The unique characteristics of Schottky junctions make them integral to various electronic devices, enabling crucial functionality in modern technology.
The performance of P-N junctions is influenced by several factors, including temperature, light intensity, and voltage, each of which plays a crucial role in shaping the junction’s electrical characteristics and operational efficiency.
Temperature exerts a significant influence on the performance of P-N junctions, affecting carrier mobility, thermal energy considerations, and the breakdown voltage crucial for the junction’s operational stability.
At higher temperatures, the carrier mobility of the P-N junction increases due to the higher thermal energy available in the semiconductor material, promoting the movement of charge carriers. This increase in carrier mobility can also lead to a higher rate of recombination, affecting the junction’s overall performance.
Elevated temperatures can cause a reduction in the breakdown voltage of the junction, impacting its ability to withstand reverse bias conditions and potentially compromising its operational integrity.
Light intensity directly influences the performance of P-N junctions through photon absorption, the generation of minority carriers, and the manifestation of the photovoltaic effect in solar cell applications.
This impact is significant as it determines the amount of energy that can be harnessed.
When light intensity increases, more photons are absorbed by the semiconductor material, leading to greater generation of electron-hole pairs. This, in turn, enhances the flow of current in the solar cell, resulting in increased efficiency.
The relationship between light intensity and the electrical output of the solar cell is crucial for optimizing its performance.
Voltage considerations significantly affect P-N junction performance, encompassing aspects such as biasing, breakdown characteristics, and the distinctions between reverse and forward bias conditions that impact the junction’s behavior.”
When a P-N junction is subject to forward bias, the voltage applied aids in overcoming the potential barrier, allowing current to flow with increased efficiency. This condition promotes the majority carrier injection across the junction, leading to reduced resistance.
Conversely, under reverse bias, the voltage acts to widen the depletion region, creating a higher potential barrier and inhibiting the flow of current. This results in a limited influence on the majority carrier movement and higher resistance within the junction.
Enhancing the performance of P-N junctions involves strategies such as careful material selection, design optimization, and the application of advanced processing techniques to refine their operational characteristics and efficiency.
Material selection plays a pivotal role in improving P-N junction performance, focusing on semiconductor considerations such as bandgap, material purity, and crystalline structure to optimize the junction’s characteristics.
When considering the bandgap, the choice of semiconductor material determines the energy required to move an electron from the valence band to the conduction band, directly impacting the operational efficiency of the P-N junction.
The level of material purity influences the presence of impurities, which can significantly affect the junction’s conductivity and overall performance. The crystalline structure of the semiconductor directly dictates its electronic properties, such as mobility and carrier density, further defining the behavior of the P-N junction.
Design optimization of P-N junctions involves refining their structural layout and interface engineering to minimize performance limitations and enhance their electrical properties for specific applications.
By carefully tailoring the doping profiles and thickness of the various layers within the junction, the efficiency and performance of semiconductor devices can be vastly improved.
Optimization also plays a crucial role in reducing recombination losses and enhancing carrier mobility, ultimately leading to more reliable and efficient electronic devices.
The introduction of advanced materials and novel fabrication techniques further contributes to the refinement of P-N junctions, allowing for the precise control of electrical characteristics and ensuring compatibility with diverse technological applications.
Advanced processing techniques such as precise doping, annealing, etching, and epitaxial growth play a crucial role in improving P-N junction performance by controlling material characteristics and interface properties.
Proper doping is essential for modifying the electrical properties of semiconductors, allowing for the creation of distinct P and N regions.
Annealing helps in reducing defects and optimizing crystal structure, enhancing the overall performance of the P-N junction.
Additionally, etching is used to remove surface layers and create specific patterns, ensuring precise alignment and functionality.
Meanwhile, epitaxial growth facilitates the deposition of high-quality semiconductor layers, enabling fine-tuning of material characteristics and optimizing interface properties.
A P-N junction is a boundary between a P-type semiconductor (with positive charge carriers) and an N-type semiconductor (with negative charge carriers). It is an essential component in semiconductors as it allows for the creation of diodes, transistors, and other electronic devices.
At the P-N junction, there is a diffusion of charge carriers from the P-type to the N-type material, creating a depletion region where there are no mobile charge carriers. This depletion region acts as a barrier for current flow, allowing for the control of electric current in semiconductors.
The main difference is the majority charge carriers present in each type of semiconductor. P-type semiconductors have positive charge carriers (holes), while N-type semiconductors have negative charge carriers (electrons). This difference is crucial in the formation of a P-N junction.
Applying a forward bias to a P-N junction means connecting the P-type side to the positive terminal and the N-type side to the negative terminal. This reduces the depletion region, allowing for current to flow. A reverse bias is when the P-type side is connected to the negative terminal and the N-type side to the positive terminal, increasing the depletion region and preventing current flow.
The P-N junction is a crucial component in diodes as it allows for the control of current flow. In a forward bias, the diode allows for current to flow, acting as a closed switch. In a reverse bias, the diode acts as an open switch, preventing current flow.
Yes, the P-N junction is widely used in electronic devices such as diodes, transistors, solar cells, and integrated circuits. It is also essential in modern technology, allowing for smaller and more efficient electronic devices.